The atomic clock greatly improved the precision of time measurement, but now research is being carried out on a new atomic clock with precision that far surpasses even that. Atoms absorb and emit not only radio waves, but also light of specific frequencies. Research aims to identify this light and use it as a base unit for time.
What is an optical (light-based) clock?
Why use light instead of radio waves? The reason for this is that light has a higher frequency than radio waves. Clocks and watches measure time based on some type of oscillation. Replacing radio waves with higher frequency light waves will lead to higher precision.
However, the problem that has faced this research is that, due to the higher frequency, the Doppler effect caused by the motion of atoms leads to variations in the timepiece. To solve the issue, two methods have been proposed for a mechanism to halt the motion of atoms and hold them in one place.
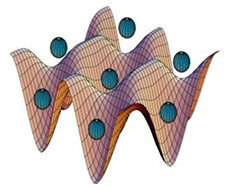
One is the ion trap. This method identifies frequency by trapping a single atom with one electron removed (an ion) in an electric field (the trap) and removing noise through multiple measurements of the ion’s resonance frequency. The drawback to this method is that it requires more than ten hours to perform measurements, as 1 million separate measurements are necessary.
The other is the “optical lattice clock,” an original Japanese method invented and developed by Professor Hidetoshi Katori at Tokyo University. Overlaying two lasers of different phases causes them to alternatively intensify and weaken each other at different points, creating “interference fringes” resembling the shape of an egg carton, as shown in the illustration. Atoms are caught in these interference fringes. By capturing and measuring many atoms at once, this system is able to reduce the time needed for measurement. The drawback to this method is the potential for deviations in frequency due to the mutual influence of many captured atoms being held in relatively close proximity to each other. If the precision of the optical lattice clock improves, it will no longer be possible to ignore the influence of the atoms on each other, and the minimization of that influence will be a topic of research for further improvement.
Atoms from Groups 2 and 3 of the periodic table, such as strontium, calcium, and mercury, and related elements, are used for either method, and competitive research using these two methods is currently being carried out at the most advanced research facilities around the world.
Japan at the forefront
A major obstacle to achieving an optical clock was that optical frequencies could not be measured accurately. This problem was solved by the invention of a mechanism called an “optical frequency comb,” which is used as a ruler for measuring light. Before the development of this tool, measurements had to be performed with a series of short rulers (radio waves), which caused significant errors in measurement, but the invention of a long ruler (the optical frequency comb) has made it possible to measure optical frequencies easily and accurately.
The mechanism of the optical frequency comb is as follows. First, light is controlled in ultrashort pulses by an atomic clock and a series of precise pulses is continually emitted at intervals about equal to a radio wave cycle. This causes the light to become an optical signal on the scale of the radio wave range at the precision of the atomic clock. By introducing various contrivances and using this precise scale as a standard, optical frequencies can be measured with the same precision as an atomic clock. This technology was awarded the Novel Prize in Physics in 2005, just five years after it was developed.
Another problem is the matter of how to verify the precision of an optical clock. As there are no clocks with higher precision anywhere in the world, it could be said that there is no “ruler” by which to verify the precision. The solution to this problem is a method of confirming precision through comparison between two high-precision clocks.
Japan has been at the forefront in experiments involving the comparison of clocks. In 2011, the National Institute of Information and Communications Technology (NICT) developed a technology to precisely transmit an optical frequency signal via optical fiber between its head office in Koganei City and the Tokyo University Hongo Campus. Through comparison of the frequencies of the two optical lattice clocks, respectively operated by Professor Hidetoshi Katori’s laboratory at Tokyo University and the NICT’s Space-Time Standards Laboratory, the two clocks were confirmed to correspond at a precision of 10-16.
In Europe, a project is currently underway to connect four laboratories in the UK, Germany, France, and Italy with optical fiber cables to confirm the precision of atomic clocks operated by each of the laboratories. In 2016, optical lattice clocks operated by SYRTE in France and PTB in Germany were connected via a 1,400 km optical fiber cable and were confirmed to have a corresponding precision of 5 x 10-17. In the United States, two laboratories in Colorado have been connected to make the same confirmation.
As research progresses, it is expected that in another 10 years the one-second standard currently based on cesium radio waves will be supplanted by an atomic optical standard.
What ultra-high-precision clocks can provide
Theoretically, an optical lattice clock has a precision of 10-18, which is equivalent to an error of one second in 30 billion years. It is thought that the earth was formed around 4.6 billion years ago and the universe was formed around 13.8 billion years ago, so 30 billion years is more than seven times the age of the earth and twice the age of the universe. How would such a high-precision clock affect our lives?
In actual fact, ultra-high-precision time measurement can lead to great advances in science. The theory of relativity developed by Einstein holds that gravity influences the flow of time. Time flows more slowly in areas of strong gravity. The ability to perform ultra-high-precision time measurement makes it possible to measure differences in gravity.
An experiment making a direct measurement of the influence of gravity has already been performed. NICT compared an atomic clock made for a long-wave radio station with Japanese Standard Time, in Tokyo, and then took the clock to a long-wave radio station at an altitude of nearly 1,000 meters. In doing so, they were able to confirm a variation in the passage of time almost exactly as predicted by the theory of relativity.
In addition, the above-mentioned frequency correspondence investigated through the optical fiber link between Koganei and Hongo was confirmed after real-time detection and correction of a time gap due to the approximately 50 m difference in altitude between the two locations.
As practical applications in other areas, ultra-high-precision clocks are also expected to significantly improve precision of positioning technologies such as GPS and vastly increase the capacity of communications technologies such as optical fibers.
We will be watching with eager anticipation to see what advances will be made in atomic clocks and what ripple effects they will have in the future.